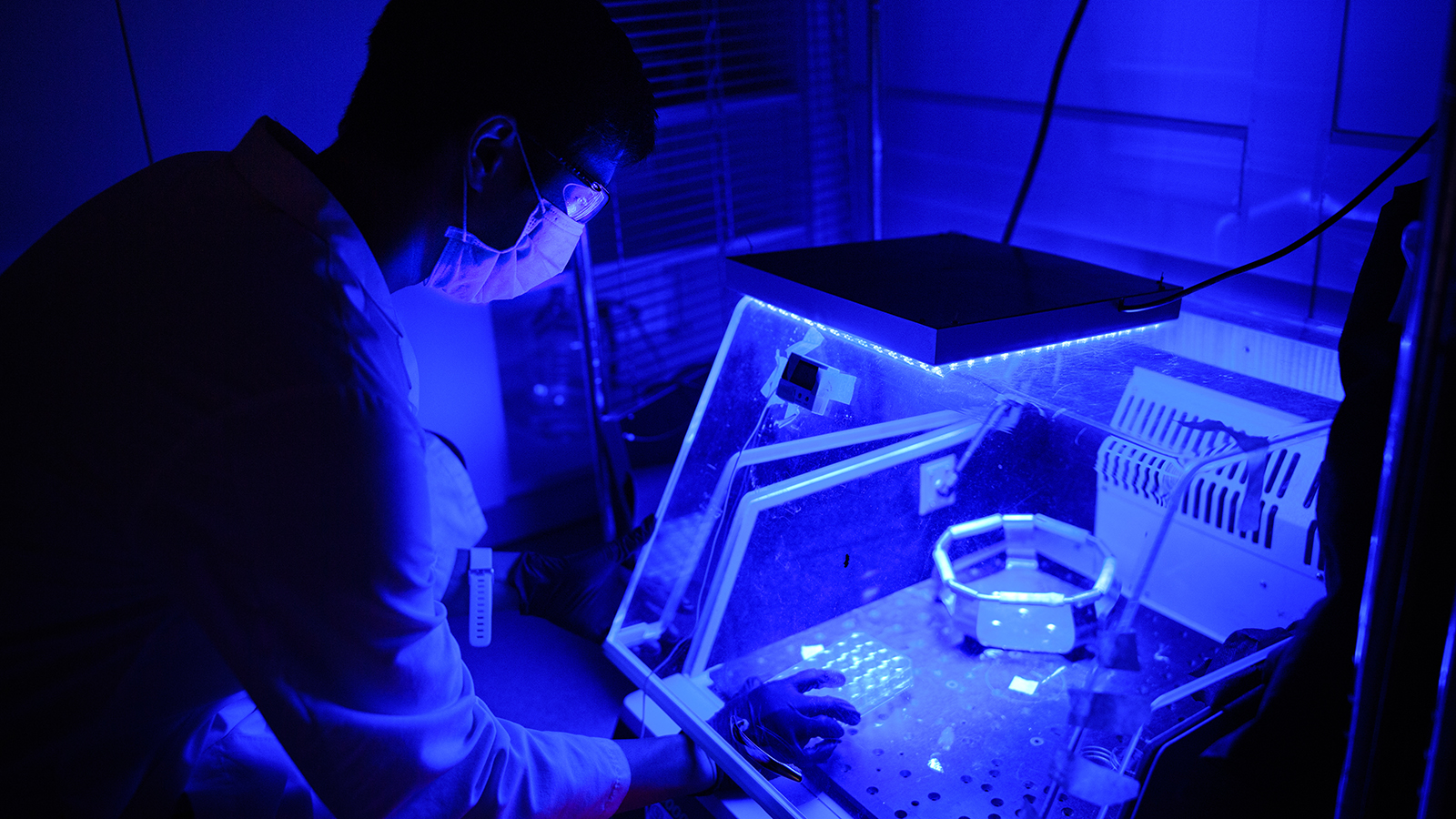
By Molly A. Seltzer
Strains of microbes like yeast and E. coli can be engineered to produce useful chemicals and fuels, and can produce more fuel more efficiently by working together. The problem is that when grown together in co-cultures, the fastest-growing strain often outcompetes the others, causing the community to break down and stop chemical production. Now, Princeton researchers have discovered a new way to stabilize co-cultures of microbes using light. By engineering the faster-growing strain to respond to light, the researchers can control its growth using light pulses, stabilizing the community as a whole and optimizing it for chemical production.
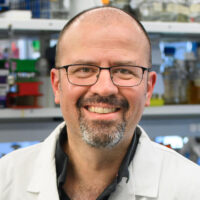
This research was led by José Avalos, assistant professor of chemical and biological engineering and the Andlinger Center for Energy and the Environment, and published in ACS Synthetic Biology. Avalos and his team developed consortia of the bacteria E. coli and yeast S. cerevisiae, in which each strain was engineered with part of the genes for the biosynthetic pathways of two chemicals. One consortium was developed to produce isobutyl acetate, an advanced biofuel, and a second consortium to produce naringenin, an anti-inflammatory.
In the first consortium, the genetically modified E. coli converts sugars to isobutanol, which is secreted into the medium. The yeast can then uptake this isobutanol and convert it to the final product of interest, isobutyl acetate.
“We’re able to harness the unique strengths of each organism. E. coli is better at producing simple building block materials and the yeast is better at converting them to complex molecules,” said Makoto Lalwani, a former graduate student in Avalos’ lab and now a research fellow in Harvard’s Wyss Institute for Biologically Inspired Engineering.
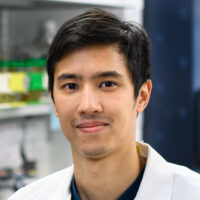
Avalos said this division of labor approach to cellular function is not new. He said that by using more than one organism, the researchers can avoid extensive genetic modifications that might compromise efficiency later. This approach reduces the burden on individual cells and allows for streamlining of metabolic processes across organisms.
“Many steps are involved in making a product of interest. If you put all the steps in one strain, you often need to make compromises in the conditions for the sake of overall efficiency. But, if you divide the steps between multiple strains it is easier to optimize each step to maximize overall productivity,” said Avalos.
The challenge, Avalos said, is that although the work is divided across two or more strains and they are each programmed to do their part of the reaction, the strains are not programmed to work together, or ensure that both thrive in the co-culture. Ordinarily, when E. coli and yeast are grown together without light controls, E. coli would outcompete the yeast because its growth rate is much greater.
According to Avalos, there have been elegant solutions proposed to overcome this challenge, such as engineering mutualisms across two species so they depend on each other. However, most strategies rely on controlling the starting population ratios, which makes it difficult to control populations — and thus optimize co-culture composition for chemical production —during the fermentation.
“Even if you change the starting population, you do not have any control over what you end with or what happens in between. So, the light provides that control in between,” Lalwani said.
Avalos said the team was not looking to achieve a specific ratio of E. coli to yeast or level of fuel production; the experiment was meant to be a proof of principle to show that optogenetics, or using light to control the expression of genes, can be used to help co-cultures grow stably at desired population compositions and improve the production of chemicals of interest.
By exposing the co-cultures to specific light pulses, the researchers identified conditions in which higher amounts of the target chemical were produced. It was not high-or low-light conditions that led to the greatest chemical production, but intermediate amounts of light exposure that created the highest amounts of the chemicals of interest. The research found the final isobutyl acetate production was 83% higher when using a light-regulated bacterial strain relative to using a light-insensitive strain.
The researchers said optogenetics has not previously been used for stabilizing co-cultures. They said that historically optogenetics has been used to study cellular processes, but that using light to control metabolism is growing in popularity.
According to Lalwani, the benefit of using co-cultures goes beyond fuel production. He said co-cultures are useful for bioremediation because multiple organisms may eat different pollutants. Co-cultures can also be useful in agricultural and food technology applications and for studying microbiomes.
“But if one strain outcompetes the other, then you don’t have a co-culture anymore. That’s a situation in which light might be useful to grow two strains in harmony so they can do their respective jobs,” said Lalwani.
The researchers said this work opens the door to using co-cultures more widely and successfully across fields.
Authors in addition to Avalos and Lalwani include Hinako Kawabe, Rebecca L. Mays, and Shannon M. Hoffman who are of the Department of Chemical and Biological Engineering at Princeton University. The work was supported by Princeton SEAS Project-X, the U.S. Department of Energy, Office of Science, Office of Biological and Environmental Research Award Number DE- SC0019363, the NSF CAREER Award CBET-1751840, The Pew Charitable Trusts, and The Camille Dreyfus Teacher-Scholar Award.