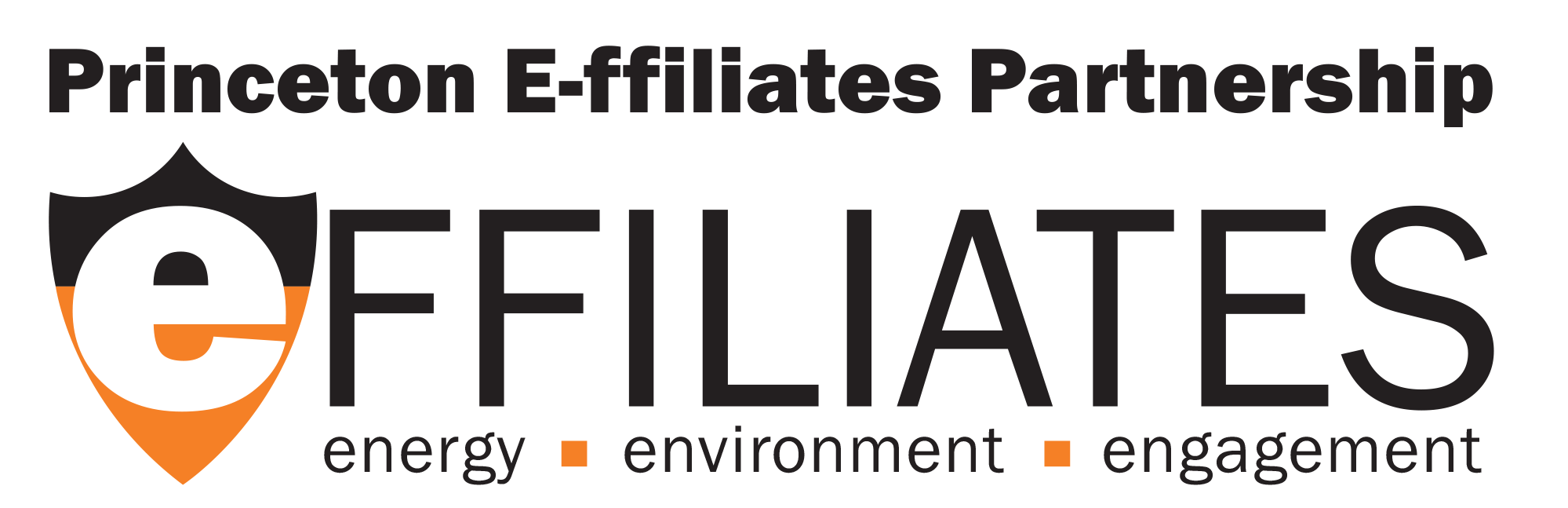
Building a clean future:
Decarbonizing cement, steel,
and other heavy industries
Posters
-
Sérgio Angulo1,3
Visiting Fellow
Collaborator: Claire White2,3With Mateus Zanovello1
1 Department of Construction Engineering, University of Sao Paulo, Brazil
2 Department of Civil and Environmental Engineering
3 Andlinger Center for Energy and the EnvironmentRecycled (Thermoactivated) Cement Waste: A Low-CO2 Circular Engineered Binder
Abstract
Yearly, 2 billion tons of old cement from concrete waste remains underutilized despite the fact that this cement can be recycled to significantly offset the CO2 emissions from cement production. In this research, we present a new low-CO2 engineered blended cement containing less than 20% Portland clinker (270-340 kg CO2/t; lower than those obtained with other scalable SCMs). By employing synchrotron XRD measurements conducted at Brookhaven National Laboratory, we show that thermoactivation of old cement can be used as a circularity path for concrete, where the main binder gel, calcium-silicate-hydrate (C-S-H), successfully reforms via hydration after each thermal cycle. From our CO2 emissions analysis, we show that the use of renewable energy or low-CO2 thermal heat (as electricity in Brazil) can cut CO2 emissions of the recycled cement by half, and, by use of CO2 mineralization process (CO2 capture), the engineered circular binder can achieve net-zero emissions, a fundamental step for the low-carbon construction industry and society.Bio
Angulo earned his Ph.D. in civil engineering from the University of Sao Paulo. He developed postdoctoral studies at Bauhaus University, Germany, the University of Illinois Urbana-Champaign, and now at Princeton University. He works as an associate professor at the University of Sao Paulo and develops research in low CO2 cementitious binder, construction waste recycling, and materials characterization. -
Mohamed Atouife1
2nd year Ph.D. candidate
Principal Investigator: Jesse Jenkins1,21 Department of Mechanical and Aerospace Engineering
2 Andlinger Center for Energy and the EnvironmentIron and Steel Decarbonization in the Inflation Reduction Act Era: Imminent Relining of Coal-based Assets and the Prospects of Renewable-Hydrogen-based Direct Reduction
Abstract
The iron and steel industry in the United States stands at a critical juncture. On one side, all blast furnace assets face imminent relining investments this decade, posing the risk of prolonging dependence on coal-based steel production assets. On the other side, renewable-hydrogen-based direct reduction (DRI) is on the verge of large-scale commercialization and clean energy incentives in the Inflation Reduction Act (IRA) strengthen the argument for transitioning to this low-carbon production pathway. In this context, we aim to evaluate whether IRA incentives suffice to ensure the economic viability of renewable-hydrogen-based steel compared to coal-based steel, thereby unlocking investment in clean steel production and averting blast furnace relining. Using an optimization model, we estimate the resource requirements (including renewable energy, DRI, electrolysis, hydrogen storage) based on the renewable energy potential in existing blast furnace locations. Additionally, we estimate the cost of hydrogen-based steel under various configurations (such as flexible sizing and operation, availability of salt cavern storage, etc.). Our analysis indicates that while IRA incentives significantly reduce the cost gap between coal-based and renewable-hydrogen-based steel, the latter remains more expensive, with a premium amounting up to 200 USD per ton of steel even after incentives. Therefore, to stimulate clean steel investments in the short term, further policy support and/or long-term demand-side offtake agreements at a premium are imperative. Looking ahead to 2030, learning-induced electrolysis cost declines driven by a successful take-off of the water electrolysis industry, combined with IRA incentives, could unlock cost parity between hydrogen-based and coal-based steel.Bio
Atouife holds a bachelor’s degree in mathematics and physics from Ecole Polytechnique, France. His research interests include energy-system modeling, emerging clean technologies, industrial decarbonization, and politically feasible pathways to net-zero. -
Sophia L. Bergen1
1st year Ph.D. candidate
Principal Investigator: Claire E. White1,21 Department of Civil and Environmental Engineering
2 Andlinger Center for Energy and the EnvironmentEvaluation of Inter-grinding to Increase the Reactivity of Abundantly Available Basalt for Sustainable Cements: Earth and Beyond
Abstract
Basalt is an abundantly available natural resource, but due to its lower reactivity in alkaline environments compared with commonplace supplementary cementitious materials (SCMs), such as calcined clays, it has yet to be extensively explored as a sustainable additive or baseline material for use in concrete. Here, we investigate the viability of increasing the reactivity of basalt via its inter-grinding with an alkali source (such as sodium hydroxide or sodium silicate) to make a “one-part” alkali-activated cement binder. The preliminary findings and characterization of raw materials will be presented, including mineralogy, calorimetry, and Fourier transform infrared spectroscopy. Moreover, due to the composition and mineralogy similarities between basalt and lunar regolith, this project also explores the development of a “one-part” alkali-activated lunar regolith simulant (LMS-1). NASA’s Artemis program is a series of lunar missions designed to return to the moon, and constructing a lunar base is critical to this program. Hence, this research underscores the importance of cross-disciplinary approaches, showcasing the transferability of innovative technologies across seemingly disparate environments, namely Earth and the Moon.Bio
Bergen earned a bachelor’s degree in environmental engineering from Cornell University. Her research interests include increasing the reactivity of alternative materials to develop novel sustainable cements. She is also interested in lunar construction materials, and how the development of these materials may be simultaneously beneficial to construction on Earth. Bergen has published in Renewable and Sustainable Energy Reviews and DEVCOM Army Research Laboratory journals. She is also a 2nd Lieutenant in the U.S. Army Reserves, serving as an Engineer Officer. Bergen looks forward to combining her passions for public service and sustainable infrastructure throughout her career. -
Xunkai Chen1
3rd year Ph.D. candidate
Principal Investigator: Kelsey Hatzell2,31 Department of Chemical and Biological Engineering
2 Department of Mechanical and Aerospace Engineering
3 Andlinger Center for Energy and EnvironmentSilicon Anode Degradation During Freeze-thaw Temperature-Swings
Abstract
Batteries used in space applications can be exposed to large temperature swings. During these large temperature swings, the battery electrolyte can undergo a phase transformation from a liquid to a solid and back to a liquid. The nature of the solvent and the type of salt influence crystallization processes. Herein, we aim to understand how pressure build-up in confined regions of an electrode (e.g. pores) influences degradation processes in silicon-oxide graphite anodes undergoing freeze-thaw dynamics. Our results show that high porosity electrodes lead to a greater density of nucleation sites for electrolyte crystallization. Local pressure buildup at pores results in active material loss and decreased cycle lifetime in batteries exposed to extreme temperature swings.Bio
Chen earned his bachelor’s degree in chemical and biomolecular engineering from the University of California, Berkeley. He has a diverse research background in theoretical modeling, 2D graphene materials, polymer/polyelectrolyte chemistry, and materials characterizations. In graduate school, he is interested in studying silicon-based anode failure mechanisms using electrochemical and x-ray imaging techniques. He hopes that by developing a comprehensive understanding of silicon-based electrode failure behaviors, he can use his knowledge to contribute to the commercialization of energy-dense and long-cycle life batteries for energy storage applications. -
Rahul Gaur1
Postdoctoral research associate
Principal Investigator: Egemen Kolemen1,2,5With Dario Panici1, Rory Conlin3, Daniel Dudt4, Y. Elmacioglu1, K. Orr1, K. Unalmis1, E.Kolemen1,2,5
1 Department of Mechanical and Aerospace Engineering
2 Princeton Plasma Physics Laboratory
3 University of Maryland, College Park
4 Thea Energy
5 Andlinger Center for Energy and the EnvironmentA Novel Optimization Tool for Finding the “Best” Stellarator
Abstract
Stellarators are toroidal nuclear fusion devices that confine a hot plasma (150 million K) using externally applied magnetic fields. The lack of a continuous symmetry in stellarators leads to huge design space (billions) of possible stellarator equilibria. Hence, finding the “best” stellarator becomes a sophisticated optimization problem. To this end, we present the DESC optimization suite, a rapidly-growing, GPU-accelerated, stellarator optimization code. We demonstrate its utility by finding stellarator equilibria with favorable properties such as simple electromagnetic coil shapes, magnetohydrodynamic (MHD) stability, and reduced turbulence.Bio
Gaur earned a bachelor’s degree in mechanical engineering from Indian Institute of Technology, Delhi and a doctorate in physics from the University of Maryland, College Park. His research interests include analysis and optimization of nuclear fusion devices for magnetohydrodynamic (MHD) stability and reduced turbulence. Gaur hopes the research will help find optimized stellarator equilibria that will lead to a viable nuclear fusion power plant. Gaur will begin his second term as a postdoctoral fellow at Princeton University in the summer of 2024. -
Tubagus Aryandi Gunawan1
Postdoctoral research associate
Principal Investigator: Chris Greig1With Fangwei Cheng1, Eric Larson1, Imam Santoso2, Sarjiya3,4
1 Andlinger Center for Energy and the Environment
2 Department of Metallurgical Engineering, Bandung Institute of Technology, Indonesia
3 Department of Electrical Engineering and Information Technology, Gadjah Mada University, Indonesia
4 Center for Energy Studies, Gadjah Mada University, IndonesiaTowards Net-Zero Indonesia: Clean Nickel Production
Abstract
Nickel is a critical metal for the energy transition. Half of all nickel ore mining and processing globally takes place in Indonesia today. In the last decade, Indonesian nickel production soared more than four times to two million tonnes per annum. With some 9 GW of coal power plants built primarily to power this growth, Indonesia’s nickel production is carbon intensive. We are developing methods & tools for assessing decarbonization scenarios and costs for nickel facilities, using Indonesia’s Obi Island as a case study. Our work includes (1) estimation of facility-level CO₂ emissions, (2) integration of CO₂ capture and storage (CCS), biomass utilization, and/or renewable power generation with nickel facilities, (3) evaluation of capital investments needed, and (4) assessment of coal power assets that could be stranded. Future work is planned to expand the analysis to all of Indonesia’s nickel production, estimating the costs of clean nickel production, and estimating industry-specific carbon abatement cost.Bio
Gunawan’s research interests include techno-economic-environmental modelling of clean energy systems, including hydrogen and electrofuel production systems, carbon capture technologies, supply-chain optimization, power and transportation sector couplings, energy storage, and renewable energy. He obtained a bachelor’s degree in chemical engineering from the University of Indonesia, a master’s degree in energy engineering from the Technical University of Berlin where he was also involved with modeling solar-based synthetic liquid fuels production at the German Aerospace Center, and a Ph.D. in mechanical engineering from the University of Galway that included developing an online decision support tool for deploying renewable hydrogen in northwest Europe. -
Shashank Gupta1
3rd year Ph.D. candidate
Principal Investigator: Reza Moini11 Department of Civil and Environmental Engineering
Tough Cortical Bone-Inspired Tubular Architected Cementitious Materials
Abstract
Cortical bone is a tough biological material composed of tube-like osteons embedded in the organic matrix surrounded by weak interfaces known as cement lines. The cement lines provide a microstructurally preferable crack path, hence triggering in-plane crack deflection around osteons due to cement line-crack interaction. Here, inspired by this toughening mechanism and facilitated by a 3D-printing as well as hybrid (3D-printing/casting) processes, we engineer architected tubular cement-based materials with a new stepwise cracking toughening mechanism, that enabled a non-brittle fracture. Using experimental and theoretical approaches, we demonstrate the underlying competition between tube size and shape on the stress intensity factor from which engineering stepwise cracking can emerge. Two competing mechanisms, both positively and negatively affected by the growing tube size, arise to significantly enhance the overall fracture toughness by up to 5.6-fold compared to the monolithic brittle counterpart without sacrificing the specific strength. This is enabled by crack-tube interaction and engineering the tube size and shape, which leads to stepwise cracking and promotes rising R-curves. Furthermore, ‘Disorder’ curves are proposed for the first time to quantitatively characterize the degree of disorder for describing the representation of architected arrangement of materials (using statistical mechanics parameters) in lieu of otherwise inadequate ‘periodicity’ classification.Bio
Gupta earned a bachelor’s degree in civil engineering from BITS Pilani, India, and a master’s degree in civil engineering (structures) from Politecnico di Milano, Italy. At Princeton, his research revolves around the design and fabrication of bio-inspired architected cementitious materials and understanding their fracture mechanics using experiment, modeling, and advanced characterization techniques. Gupta hopes that his research will aid in enhancing the crack-resistant characteristics in brittle and quasi-brittle cementitious materials through deliberate designs at meso-scale. Gupta’s work has been accepted in Advanced Functional Materials and he has published in Cement and Concrete Composites in addition to co-authoring 11 peer-reviewed papers in the field of cementitious materials. -
Yuezhang He1,3
3rd year Ph.D. candidate
Principal Investigator: Jesse Jenkins1,2With Zheng Li3, Pei Liu3
1 Department of Mechanical and Aerospace Engineering
2 Andlinger Center for Energy and Environment
3 Department of Energy and Power Engineering, Tsinghua University, ChinaDecarbonization Pathways and Layout Evolution in China’s Steel Sector
Abstract
Decarbonizing the iron and steel sector is crucial for global climate goals. Downscaling decarbonization should be explored for responsibility allocation and low-carbon technologies. Accurate emission reduction assessments must consider spatiotemporal industrial layout evolution driven by technology and demand trends. This study uses optimization modeling to analyze the decarbonization pathway, considering industrial layout changes. An integrated model for demand forecast, technology selection, and inter-provincial transport was developed. In China, steel production may shift from North to East, Southwest, and South China, improving provincial self-sufficiency. Deep emissions reduction requires secondary routes and primary routes with CCS or H2-DRI technologies.Bio
Yuezhang earned a bachelor’s degree in the Department of Energy and Power Engineering at Tsinghua University. He is now a visiting Ph.D student at Princeton University to conduct joint research between Princeton and Tsinghua. He is strongly interested in climate change mitigation and energy transition. His research interests include industrial sector decarbonization and supply chain analysis in the energy system, based on optimization approaches. As a youth delegate concerned about climate change issues, Yuezhang has attended COP27, the Global Youth Summit on Net-zero Future, COP15, and the Student Energy Summit. -
Michael C. James1
Postdoctoral research associate
Principal Investigator: Claire E. White1,2With Matthew J. Pekarcik, Jr.3, David B. Graves 3,4
1 Andlinger Center for Energy and the Environment
2 Department of Civil and Environmental Engineering
3 Department of Chemical and Biological Engineering
4 Princeton Plasma Physics LaboratoryCa-based Layered Double Hydroxides for CO2 Capture
Abstract
Layered Double Hydroxides (LDHs) and LDH-derived mixed metal oxides (MMOs) are of interest for CO2 capture applications as they have large surface area, high selectivity for CO2 sorption, and are cheap to produce. LDHs show good CO2 capture performance under high humidity conditions, due to interaction between CO2 and H2O before reacting with LDH to produce calcite. By contrast MMOs, produced by calcining LDHs, physisorb CO2 in a reversible process. This project investigates the use of low temperature plasmas for energy-efficient CO2 capture and removal from these sorbent materials.Bio
James obtained his undergraduate master’s degree in chemistry and his Ph.D. in functional nanomaterials from the University of Bristol (UK). He then held two postdoctoral research associate positions at Bristol, before working in research funding for several years for the UK’s Engineering and Physical Sciences Research Council (EPSRC). James’ research interests include developing nanomaterials for CO2 capture and renewable energy generation. -
Debra A. Keiser1
4th year Ph.D. candidate
Principal Investigator: Claire E. White2,31 Department of Chemistry
2 Department of Civil and Environmental Engineering
3 Andlinger Center for Energy and the EnvironmentApplication of a Structural Descriptor and Unsupervised Learning Algorithms to Analyze Molecular Models of Disordered Calcium Silicate Hydrate Gels
Abstract
Replacing ordinary Portland cement with supplementary cementitious materials (SCMs) reduces the cement industry’s carbon footprint. However, doing so alters fundamental properties of calcium silicate hydrate (C-S-H) gel, the main strength-giving component of concrete. This may impact concrete’s long-term durability and serviceability. Here, force field molecular dynamics simulations have been conducted to understand how SCMs affect the structure of C-S-H gel. Atomic descriptor, dimensionality reduction, and clustering algorithms were employed to analyze models of C-S-H and calcium sodium aluminosilicate hydrate (C-(N-)A-S-H) gels. Results provide valuable insight into the unique chemistries of and local environments that exist within these inherently disordered phases.Bio
Keiser earned her bachelor of science in chemistry from Stony Brook University, which is also where she developed a passion for computational chemistry. At Princeton, she is interested in utilizing molecular modeling methods to study the chemistries of cementitious gels and ion interactions at gel surfaces. Keiser aims to facilitate the formulation of sustainable cement that will improve concrete’s resistance against chemical attack. She intends to pursue a career in industry designing environmentally friendly materials after she graduates next year. -
Yangwoo Lee1
1st year Ph.D. candidate
Principal Investigator: Claire White1,21 Department of Civil and Environmental Engineering
2 Andlinger Center for Energy and the EnvironmentMolecular Dynamics Simulations of Sodium-Alumino-Silicate-Hydrate Gel:
Sustainable Cements for Enhanced Geothermal SystemsAbstract
Geothermal energy, recognized for its potential to mitigate global warming through carbon-neutral solutions, demands durable infrastructure. Well cementing techniques, in particular, must be durable to ensure long-term cost-effectiveness and simultaneously, the cement used must be eco-friendly to minimize the carbon footprint and achieve carbon-neutrality. Low-Ca alkali-activated materials (AAMs) emerge as a promising candidate for this, offering a sustainable alternative to conventional cement with lower environmental impact. This project explores the formation and molecular structure of sodium-alumino-silicate-hydrate (N-A-S-H) gels, a primary component of low-Ca AAMs, under geothermal and ambient conditions. By leveraging molecular dynamics (MD) simulations, we analyze the gelation process of N-A-S-H gels under high temperature and pressure characteristic of geothermal environments. These findings are pivotal for the development of specialized cement formulations tailored for geothermal energy extraction.Bio
Lee is a 1st year Ph.D. student in the Sustainable Cement Group led by Professor Claire White in Civil and Environmental Engineering and Andlinger Center for Energy and the Environment. He earned both his bachelor’s and master’s degrees in civil and environmental engineering from Seoul National University. His research interests lie in addressing sustainability issues within the cement industry through advanced molecular simulations including reactive molecular dynamics and density functional theory. Currently, he is focusing on developing sustainable cement for geothermal conditions. Lee has contributed to four co-authored papers in the cement chemistry field. -
Sidan Lu1
Postdoctoral research associate
Principal Investigator: Z. Jason Ren1,2With Allyson McGaughey4, Sungju Im3, Yiming Liu3, Xinyi Wang3, Aaron Leininger1,
David Jassby3, Eric Hoek3, Z. Jason Ren1,21 Department of Civil and Environmental Engineering
2 Andlinger Center for Energy and the Environment
3 Department of Civil and Environmental Engineering, University of California
4 Department of Civil, Construction and Environmental Engineering, University of New MexicoMembrane Electrolysis Distillation (MED) for Volatile Fatty Acids Separation from pH-neutral Wastewater
Abstract
Anaerobic Digestion (AD) offers a robust strategy for wastewater-based chemical recovery. As global decarbonization goals intensify, there’s a mounting emphasis on amplifying Volatile Fatty Acid (VFA) yields while curtailing methane generation. To both mitigate product inhibition and bolster acid production, direct VFA extraction from anaerobic reactors is pursued. Nonetheless, in pH-neutral wastewater, VFAs predominantly exist in an ionized state, complicating molecular-form evaporation extractions. Here, we introduce a pioneering technology named as membrane electrolysis distillation (MED). This innovative system combines joule heating with pH modulation in one single membrane to enable efficient VFA extraction from pH-neutral wastewater. The results demonstrated the acid flux rate of 12.03 g/m2/h with little energy input, comparable to that using methods such as vacuum membrane distillation employing acidic solutions. Furthermore, a physio-chemical based model was developed to estimate the pH of the membrane surface and the impacts of the heating temperature. The results showed that the pH of the membrane surface was decreased to ~2.0 using the membrane electrolysis distillation, ensuring >99% of acetic acid (vs. 0.0% without pH swing) in molecular-form to be vapored upon heating. Also, the higher joule-heating temperature improves acid flux but has negative effects on the acidification. This novel system represents a promising breakthrough in the domains of sustainable resource recovery and wastewater treatment, offering a sustainable and economically viable solution to mitigate climate change and reduce carbon emissions.Bio
Lu earned her doctoral degree from Louisiana State University in 2021, specializing in environmental engineering and science. Her research focuses on resource recovery from wastewater using electrochemical and biological approaches, integrated with renewable energy sources. Lu is currently working with Dr. Jason Ren at the Andlinger Center for Energy and the Environment at Princeton University. She has developed a strong interest in wet mass biorefinery and membrane technologies for chemical separation and extraction. Through her research, Lu aims to develop more sustainable methods to support the circular carbon economy. Starting in July 2024, Lu will continue her postdoctoral training at Yale University. -
Aimane Najmeddine1
Associate Research Scholar
Principal Investigator: Reza Moini1With Shashank Gupta1
1 Department of Civil and Environmental Engineering
Phase-field Cohesive Zone Crack Propagation Model for Hard-soft Architected Materials
Abstract
Architected materials have shown promising applications across various industrial fields such as civil, aerospace, and biomedical engineering. However, these materials exhibit complex failure mechanisms, such as crack deflection, penetration, and bridging, due to the often heterogeneous or non-uniform arrangement of materials of different constitutive properties. These mechanisms are crucial in determining key bulk characteristics like fracture toughness and strength in these composite materials. One important challenge in capturing fracture behavior in soft-hard assemblies is the existence of interface regions that require special considerations in numerical simulation. To address this challenge, we present a numerically robust constitutive framework for modeling fracture behavior in architected materials composed of alternating soft and hard layered assemblies. The framework utilizes the phase-field approach to capture crack propagation within the bulk of the hyperplastic soft and elastic hard materials. Furthermore, to understand the contribution of the interface to the fracture energy potential, the constitutive relationships are further supplemented with a Cohesive Zone Model (CZM). Through a series of theoretical, analytical, and experimental evaluations, we demonstrate that our coupled phase-field CZM framework allows for the prediction of complex cracking phenomena in architected multi-materials (e.g., deflection, penetration, bridging).Bio
Najmeddine is an associate research scholar in the AM2 lab, led by Professor Reza Moini in the Department of Civil and Environmental Engineering. He earned his bachelor’s, master’s, and doctoral degrees in civil engineering from Virginia Tech in 2017, 2019, and 2022, respectively. His research interests include understanding the fracture mechanics and multi-physics of bio-inspired architected materials. His work aims to aid in the design of novel materials that can be scaled up for more resilient civil infrastructure applications. Najmeddine collaborates with researchers in the Department of Chemical and Biological Engineering and has published in various research fields, including civil engineering, computational mechanics, and fracture mechanics. -
Mansha Seth-Pasricha1
Associate Research Scholar
Principal Investigator: José Avalos1,2,4
With Jordan Mravca-Bailey2,3, Claire White2,3
1 Department of Chemical and Biological Engineering
2 Andlinger Center for Energy and the Environment
3 Department of Civil and Environmental Engineering
4 Omenn-Darling Bioengineering InstituteUtilization of an extremophilic microbe for magnesium mobilization from olivine to create a primer for carbon capture
Abstract
As global atmospheric carbon-dioxide levels have reached a record high of 417.06 parts per million (ppm) in 2022 per NOAA, among other measures, it is imperative that viable above-ground carbon storage materials and processes are developed if we are to sufficiently limit global warming. In this work we lay the groundwork for the development of a novel additively manufactured net negative CO2 biocement that will be applied to synergistic engineering, materials science, biotechnology, behavioral economics and decision theory research approaches. This carbon storage material will not only serve as a permanent sink for CO2 but will also help supplant Portland cement, currently responsible for ~8% of anthropogenic CO2 emissions. As first steps, we utilize an extremophilic microbe to induce dissolution of a non-carbonate raw material, namely olivine ((Mg,Fe)2SiO4) to release magnesium, enabling it to be a primer for carbonation reactions to precipitate Mg-carbonate phases that are responsible for formation of the solid cement material.Bio
Seth-Pasricha earned a bachelor’s degree with honors in biochemistry and a Ph.D. in microbiology and molecular genetics. Her research interests include exploring early-evolved microbes, understanding their evolutionary trajectories, and utilizing their metabolic potentials to address problems in sustainable energy, the environment, and human health. Seth-Pasricha has worked with early evolved microbes to understand evolution of cell death proteases in her Ph.D., on the evolution of double-stranded RNA bacteriophages in her postdoc, and in reproductive medicine and hereditary cancer in the precision medicine industry. At Princeton, she is collaborating with researchers in the Department of Civil and Environmental Engineering and the Andlinger Center on various projects for PFAS bioremediation, production of a microbially-manufactured net negative CO2 Biocement, and biosorption of metal ions from industrial wastes. She is excited to co-lead a new collaboration at Princeton University that connects biological and environmental engineering with science communication and visual media to engage diverse communities with the ecological ubiquity of forever chemicals. -
Meddelin Setiawan1
1st year Ph.D. candidate
Principal Investigator: Claire E. White1,21 Department of Civil and Environmental Engineering
2 Andlinger Center for Energy and the EnvironmentToward One-part Alkali-activated Metakaolin via Inter-grinding: Assessing the Effects of Inter-grinding on Reaction Kinetics and Microstructure
Abstract
Various alkali-activated materials (AAM) have been developed to help reduce carbon emissions of the construction industry. However, the commercialization of AAMs has been relatively slow, first due to a lack of widespread awareness of the emissions associated with the cement industry, and second due to the reluctance to work with “two-part” concretes, such as AAMs, that require large amounts of high pH activator solutions. Designing AAM starting materials that resemble ordinary Portland cement (OPC), where only water is needed, will potentially accelerate the implementation of this lower-CO2 cement in engineering applications. Here, we will present preliminary findings on a “one-part” AAM synthesized using abundantly available calcined kaolin clay and solid sodium sources (sodium hydroxide and sodium silicate). The effects of inter-grinding the calcined clay with the different types of sodium sources are assessed using isothermal calorimetry (reaction kinetics), Fourier-transform infrared spectroscopy (microstructure), compressive strength testing, and workability. It is anticipated that inter-grinding will aid in the reactivity of the overall system, helping to overcome the known reactivity limitations of current “one-part” AAMs.Bio
Setiawan earned a bachelor’s degree in materials science and engineering from Pennsylvania State University. She is currently working on designing ready-to-use metakaolin-based cement using her knowledge in material characterization and property control. Setiawan cares deeply about the decarbonization of the concrete industry, thus she is also aiming to investigate the policies and/or standards that will aid the process. Setiawan is expecting a paper about High Energy X-ray Diffraction Microscopy (HEDM) methodologies to be published and to complete her graduate degree in civil and environmental engineering by 2028. -
Anita Zhang1
3rd year Ph.D. candidate
Principal Investigator: Claire E. White1,21 Department of Civil and Environmental Engineering
2 Andlinger Center for Energy and the EnvironmentEffects of Calcium Hydroxide and Magnesium Oxide in Alkali-activated Metakaolin with Low Activator Concentrations
Abstract
Alkali-activated metakaolin (AAMK), a product of combining calcined kaolinite clay with an alkali activator such as a sodium silicate solution, is a promising type of sustainable alternative cement that emits 40-80% less CO2 than ordinary Portland cement. Currently, in a standard AAMK mix design, most of its remaining emissions come from the activator, so lowering the alkali concentration of the activator can significantly further reduce AAMK’s carbon footprint. However, the question remains as to what can be added to mix designs with low activator concentrations to minimize both short-term and long-term performance loss without significantly increasing the carbon footprint. In this study, we show that two cation sources, calcium hydroxide (Ca(OH)2) and magnesium oxide (MgO), may help offset the performance loss associated with lower activator concentrations. The pore size distributions and intrinsic permeability values of a standard AAMK mix design and various low-activator-concentration formulations were quantified using a variety of techniques. Data showed that both cation sources have notable beneficial effects on the low-activator-concentration AAMK system. The mechanism through which they helped was then studied using Fourier transform infrared spectroscopy and isothermal conduction calorimetry. Findings from our work can aid in the design and optimization of future AAMK formulations to achieve both low CO2 emissions and good performance properties.Bio
Zhang graduated from Cornell University with a bachelor’s degree in December 2020, majoring in civil engineering and minoring in applied math. Before Princeton, she worked at a sustainability-minded civil engineering B-Corp, Taitem Engineering, in Ithaca, NY and completed two internships with Arup’s Advanced Technology and Research team in New York City. At Princeton, she focuses on understanding and optimizing the durability of alkali-activated metakaolin using a variety of material characterization techniques. She is also the recipient of the High Meadows Environmental Institute’s Science, Technology, and Environmental Policy fellowship. She is currently working on her first manuscript, and she hopes her work can lead to wider adoption of sustainable cement.