New Light: Rising Stars
in Energy and the Environment
Andlinger Center 2023 Summer Seminar Series
July 19 Speakers
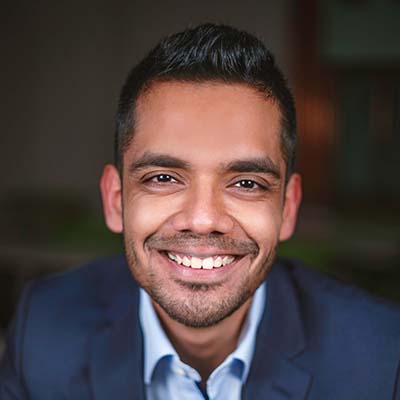
Aniruddh Mohan
Andlinger Center, Advised by Eric Larson and Jesse Jenkins
Electricity System Impacts of Direct Air Capture Deployment
Abstract
Direct Air Capture (DAC) of CO2 is receiving increased attention due to voluntary corporate procurement of DAC based CO2 removals and new subsidies for DAC in the Inflation Reduction Act. DAC is energy intensive, given the low concentration (<0.1%) of CO2 in ambient air, but offers relatively strong verification of removals and limited land constraints to scale. Here, we use the GenX capacity expansion model to study how deployment of DAC impacts the electricity system. DAC deployment will interact with the electricity system by demanding grid power, coupling with zero carbon generators such as nuclear and geothermal energy for heat which might impact their competitiveness, and potentially providing CO2 removal as a service for power sector decarbonization goals. While previous literature has analyzed DAC at the site level, we model system level impacts that might result from both near term and large-scale DAC deployment. With high uncertainty on the performance characteristics of a future DAC system, we also identify regions of the design space that offer the most value for system level CO2 reduction goals. Finally, we evaluate the cost targets necessary for DAC deployment at million-ton scale as part of achieving net-zero CO2 emissions in the electricity sector.
Bio
Mohan holds a PhD in Engineering & Public Policy from Carnegie Mellon University. He is currently a Distinguished Postdoctoral Fellow at the Andlinger Center for Energy and the Environment working with Eric Larson, Senior Research Engineer at the Andlinger Center, and Professor Jesse Jenkins to study the system-level impacts of emerging technologies in deep decarbonization pathways. This research involves applying rich geospatial datasets and modelling tools, such as optimization and agent-based modelling, to understand the application space for new technologies to help address environmental challenges.
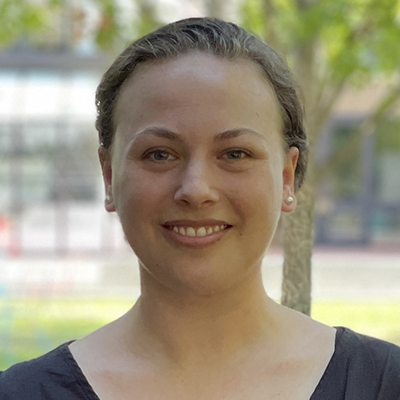
Lara Tomholt
Andlinger Center, Advised by Forrest Meggers and Reza Moini
Evaporative Cooling of Façades with Cement-based Architected Materials: Opportunities, Challenges, and What We Can Learn from Nature
Abstract
Thermoregulation of buildings is energy intensive and is a significant contributor to climate change. In turn, due to rising temperatures, the use of air conditioning is expected to surge. To address the needs of both mitigating climate change and adapting to its effects, we urgently need to develop new solutions to efficiently heat, and especially cool, our buildings. Our research explores the energy benefits of evaporative cooling of facades and how novel, architected cement-based materials provide an opportunity to maximize cooling performance. This talk highlights the design parameters that govern evaporation rate, with a particular focus on one of them: wetted surface area. Drawing inspiration from strategies employed by nature to transport fluid across large surface areas for evaporation, two distinct architected materials were developed that demonstrate considerable fluid transport capabilities, and consequently, cooling potential. Opportunities to fabricate these architected materials using modern fabrication techniques, including 3D-printing of cement paste, as well as their inherent challenges, will be discussed.
Bio
Tomholt holds a Doctor of Design (DDes) degree from Harvard Graduate School of Design. She is currently a Distinguished Postdoctoral Fellow at the Andlinger Center for Energy and Environment, working with professors Reza Moini and Forrest Meggers. Her research bridges science, technology, and design, and focuses on biologically inspired concepts for building façades with tunable heat transfer capabilities. At Princeton, Tomholt is exploring architectural design rules of biological heat management systems to develop novel 3D-printed ceramic systems with energy-efficient heat transfer for building thermoregulation.
As a Distinguished Postdoctoral fellow at the Andlinger Center for Energy and Environment, Tomholt works with professors Reza Moini and Forrest Meggers to explore novel solutions for 3D-printed ceramic systems with energy-efficient heat transfer for building thermoregulation, inspired by architectural design rules of plant leaves.
July 12 Speakers
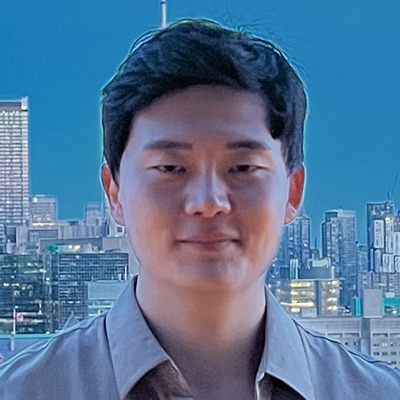
Jaewan Jang
CBE, Advised by José Avalos
Shedding Light on Microbes: Multichromatic Optogenetics for Dynamic Control in Metabolic Engineering
Abstract
Metabolic engineering is a powerful approach that enables the sustainable production of valuable chemicals, drugs, or biofuels from low-cost renewable substrates by re-wiring microbial metabolism. However, placing numerous metabolic pathways into one microbial strain poses challenges, leading to lower productivities due to impaired cell growth, suboptimal expression/activity of heterologous enzymes, and endogenous regulatory mechanisms. These challenges are addressed by forming microbial co-cultures (or consortia), in which different strains of bacteria or yeast create shared biosynthetic pathways, each producing unique intermediates. This approach significantly reduces the metabolic burden while harnessing unique capabilities of various microbial species. However, stabilizing and controlling the composition of microbial consortia is challenging due to discrepancies in growth rates between members. In the first part of the talk, the concept of using light as a noninvasive stimulus for dynamic control microbial population ratios in co-cultures will be introduced. Specifically, I will explore the ability to control the population of multiple members within consortia using different colors of light. The second part of the talk will focus on the application of optogenetics in a nonconventional yeast while highlighting its potential to produce unique chemicals.
Bio
Jang earned his Ph.D. in chemistry from the University of Toronto in 2022. He is currently a chemical and biological engineering postdoctoral fellow, working in the Avalos group. His current interests lie at the interface between optogenetics and metabolic engineering, where he aims to apply optogenetics in nonconventional yeasts to harness their unique potential for chemical production, which is lacking in conventional S. cerevisiae. In addition, he plans to employ red and near-infrared light to control populations of yeast and bacteria in microbial co-cultures for the production of valuable chemicals.
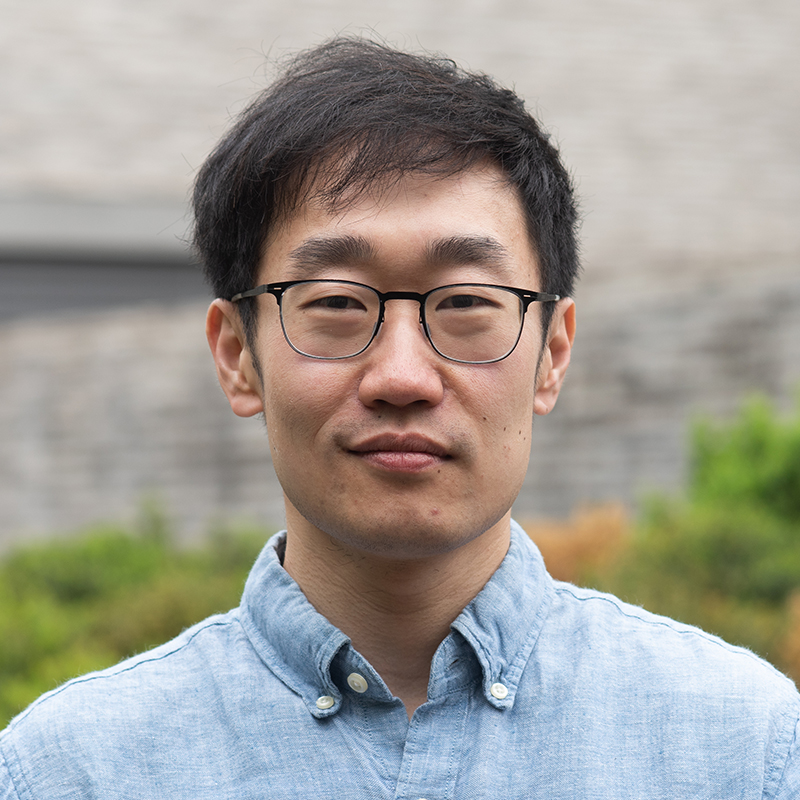
Yaguang Zhu
MAE / Andlinger Center, Advised by Kelsey Hatzell
Understanding the Critical Role of Anions in Moisture-swing CO2 capture
Abstract
Direct air capture (DAC) is essential for achieving net-zero greenhouse gas emissions by 2050. Current DAC technologies use heat or pressure to release the CO2 and regenerate the absorbent, requiring 4 to 12.5 GJ per ton of CO2. In order to overcome this extensive energy cost, a novel process using anion exchange resin to reversibly capture/release CO2 by tuning moisture levels has been proposed. In this moisture-swing DAC (MSC) process, anions undergo hydrolysis to generate hydroxide ions which react with CO2 and capture it under dry conditions. Applying water can then allow the resin to release CO2 because the reaction will proceed in the reverse direction when the humidity is high. In this talk, we evaluated the host-guest-water interactions at three length scales to help design better materials for MSC. We revealed that anion species, resin structures, and anion-resin interactions could separately affect the capacities and kinetics of the MSC process. We expect this work will pave the way for developing sustainable and less energy-demanding DAC techniques for mitigating climate change.
Bio
Zhu earned his Ph.D. in 2022 from the Department of Energy, Environmental & Chemical Engineering at Washington University in St. Louis, where he studied the nanoscale interfacial control of ion behaviors and CaCO3 formation in environmental systems using advanced X-ray and Raman characterizations. At Princeton, he is a postdoctoral research associate at the Andlinger Center for Energy and the Environment, working with Professor Kelsey B. Hatzell on novel materials to address climate change and environmental problems, with a particular focus on direct CO2 capture from air using a moisture-swing process and ion separation with MXene membranes.
June 28 Speakers
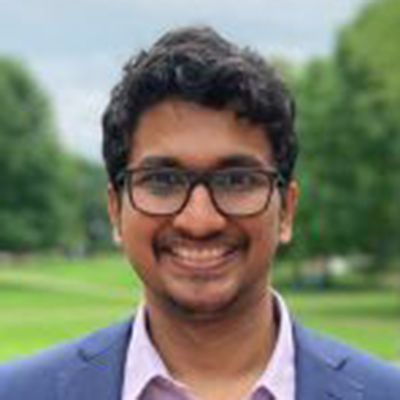
Aditya Aiyer
MAE, Advised by Michael Mueller
Harnessing the Wind (and Waves): Exploring Offshore Wind Energy
Abstract
Offshore wind energy is a rapidly growing field, with significant potential to provide clean and renewable energy. However, the operation of wind farms is challenging due to the harsh and complex offshore environment. Significant knowledge gaps exist regarding the coupling between waves, the atmospheric boundary layer, and their effects on offshore wind turbine peformance. Existing methods to quantify momentum exchange at the air-sea interface in flow simulations rely on empirical approaches that cannot be generalized to different wave conditions. I will present a new “wall” modeling approach that overcomes these limitations and accurately predicts the wind-wave momentum transfer in realistic oceanic conditions. A key advantage of the approach is that the momentum transfers due to the waves that cannot be resolved in flow simulations are calculated dynamically based on a self-consistency condition of the total drag. Finally, I will discuss the application of the dynamic wave model to offshore wind farm simulations and illustrate how it is orders of magnitude faster than alternative “brute force” simulation strategies.
Bio
Aiyer earned his Ph.D. in 2020 from the Department of Mechanical Engineering at Johns Hopkins University, where he studied the effects of microscale droplet mechanisms and ocean turbulence on the fate of oil from the Deepwater Horizon spill. At Princeton, he is a postdoctoral research associate in mechanical and aerospace engineering, working with Professor Michael Mueller on understanding processes in the marine atmospheric boundary layer that are critical for determining how much energy can be harvested by offshore wind turbines and farms.
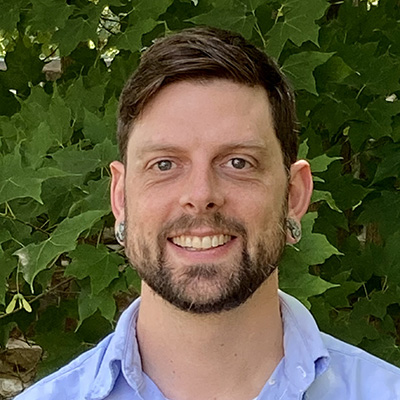
Nate Adelgren
CBE / Andlinger Center, Advised by Christos Maravelias
Three-dimensional Packing: From Offline Container Loading to Real-time Luggage Loading
Abstract
In this talk we consider the problem of determining an appropriate packing location for each of a set of rectangular items within one or more rectangular containers. Interest in this problem has grown over the past several years, due in part to the rising fuel costs, shipping container shortages, and limited workforce availability that were experienced during the COVID-19 pandemic. During the first portion of the talk we discuss several practical, real-world constraints that one may wish to impose when considering this problem, and we present techniques for reducing the computational burdens that arise from doing so. During the second half of the talk we shift our focus to the real-time loading of air travelers’ luggage. Currently, human workers are tasked with placing travelers’ luggage into unit load devices (ULDs) that are ultimately loaded onto airplanes. In order to increase efficiency and reduce workplace injuries, there is a need for this process to be automated, but doing do comes with many challenges. We discuss these challenges and propose approaches that can be used to account for them. Results are presented that demonstrate the viability of our proposed methodology.
Bio
Adelgren earned his Ph.D. in mathematical sciences from Clemson University in 2016 and subsequently held a faculty appointment at Edinboro University of Pennsylvania until 2021. He is an Associate Research Scholar in chemical and biological engineering at Princeton, working in the Maravelias Group. His research is in the general area of mathematical optimization, with current projects focused on developing improved mathematical models and related solution approaches for problems in chemical production scheduling and three‐dimensional packing.